
Owen Mumford has developed UniSafe, a springless, passive safety device designed to work with standard, prefillable syringes
According to Euromonitor International, in 2016 the value of medical device production was forecast globally to record strong growth of almost six per cent, the equivalent of approximately $315 billion. The demand for medical devices is on the increase whilst the marketplace is full of competing businesses.
As a result, there is a growing pressure on designers and manufacturers to achieve a market ready device in an efficient and timely manner. The device must also meet the brief specification, market regulations and enduser requirements. The reality is not always as straight-forward and steps must be taken to simulate or predict the performance of a device ahead of production.
At Owen Mumford, we understand that working with an array of different materials and design specifications can present challenges, so research and analysis work is imperative in ensuring we get the device design right first time.
To reduce the risk of incomplete designs or incompatible materials, it is important to combine manufacturing and design in product creation and development. This integrated approach can make all the difference.
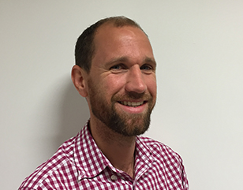
Ian Mathews, design engineering team manager R&D at Owen Mumford
Before development tooling, software simulation should be in place to analyse and optimise a design. In addition to this, rigorous testing must be undertaken to ensure that the product will perform as required during manufacture and when received by the end user. Timescales can be tight and so forward thinking alongside data analysis is key in defining the appropriate design.
There are multiple methods of simulation we use to test the performance of the design between early concept stages through to fine tuning ahead of prototyping. Five frequently used methods include:
Stress analysis is used to determine how much an element is going to be stressed or fatigued under load. Utilising Finite Element Analysis (FEA) we simulate a load on a component and discover which elements of it are undergoing high areas of stress and strain. This can also simulate the elastic element of the material and where it may potentially fail.
Optimisation systems include a range of computer systems and equations used to determine the best combination of design parameters to apply to a real-world design.
Statistical analysis uses methods such as tolerance analysis, probability simulation (for example Monte Carlo) and DOE (design of experiments). These techniques are used as part of a risk analysis approach to optimise designs and assess the impact of risk.
Mould flow is typically used to inform how to manufacture the device. We use a computer-based system to simulate what will happen when molten plastic is injected into a tool. The process evaluates the plastic flow, where it will cool and where the component’s stress points lie. This allows for many iterations to be made ahead of manufacturing.
CpK or process capability is used to understand variation within the manufacturing process. Process capability ensures consistency of all production parts throughout manufacture. Statistical methods are used to monitor this process. Variation can be caused by part design, tool design, material, machine settings, the assembly process and environmental factors such as humidity, temperature and moisture. All of these factors require consideration to determine how capable the part is.
Alongside methods of simulation, we work closely with our manufacturing teams to discuss production techniques which may help or hinder the design to manufacture process.
The simulation techniques we use are constantly evolving. As we do more simulation analysis we become better at evaluating early designs and knowing what will and will not work. This testing provides better guidance on potential issues ahead of commitment to physical prototyping.
However, there are also additional and external variables that can impact the design and must be taken into account throughout the design process. Each of these in turn can have an impact on production timelines and can include:
Clinical trials which are used to examine and evaluate both safety and efficacy of a device. One common cause of a delay or failure in clinical research is based on the inability to meet predetermined criteria and timelines set by the regulatory board, whilst other failures are due to lack of efficacy.
At this point, the product design must be reviewed and the product lifecycle has to start again. There are two kinds of trial.
The first, ‘formative’ allows us to learn how the design interacts with users and obtain feedback from a trial group, making multiple iterations along the way if required. The second, ‘summative’ is the final version of the product before sharing onwards with regulatory bodies.
Market and industry regulations which all designs must comply to. Regulatory bodies require detailed product information and have strict timelines to work with. If either of these are not met, further delays can occur as the device cannot move on to the next stage of development.
Competitor activity / new entrants to market– as with any lapse in production timeline, there is the risk of losing out to a competitor.
A release of a similar product may occur whilst adjustments are being made to the product yet to be launched. This can present its own economic pressures.
With more experience gained and more data captured from past results, designers and their manufacturing counterparts can quickly get to a stage where these tools are used to optimise rather than inform the basis of the design. Based on this experience a more productive and thorough supply chain can be achieved.
Designers and engineers must consider the challenges that lie ahead and prepare for them accordingly. This includes analysing and testing the design rigorously, working closely with regulatory bodies on the requirements needed as well managing customer expectations with what can and cannot work.
Owen Mumford on medical device manufacturing
Default