Dawn Danby and Jeremy Faludi explore the value of integrating Whole Systems Thinking into the core of your design methodology at the most formative stages
The increasing global demand for energy over the past several decades has put ever-increasing pressure on identifying and implementing ways to save energy. In response, there have been consistent improvements to energy efficiency measures worldwide.
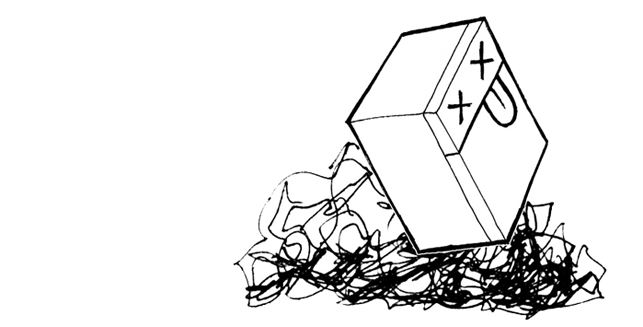
However, as successful as some energy efficiency measures have been, they’re not doing enough to keep our worldwide energy consumption levels from steadily rising. What’s gone wrong? One explanation would be that engineers are still designing for short-term energy gains, instead of keeping the whole system in mind and designing for maximum long-term energy efficiency gains.
For example, as car engines have gotten more efficient, our heavier cars force them to work harder. As our computers get faster, we load them up with more code. Even in the case of household items like refrigerators, as we make them more energy efficient, we keep plugging in more of them. It’s not unusual to find an empty fridge in every hotel room, or an extra one sitting unused in a North American garage struggling to stay cool against the summer heat. In every case, a lot of good engineering is wasted by solving the wrong problems.
Engineering alone unfortunately won’t prevent rebound effects like these or solve the global energy crisis, but designing for radical efficiency gains can help transform the ways we consume energy and slow down the rising energy consumption rates. To do this, engineers and designers need to take a look at the whole system for which they’re designing.
Thinking in systems
Does a car need motors for power-seating or does it need to burn less fuel? Even with well-intentioned innovation, it’s not unusual for an engineer to do a great job optimising a component, only to have the product as a whole perform poorly. For instance, a refrigerator might have a highly efficient compressor that costs twice as much as a normal unit, but if the fridge doesn’t seal well, a few cents worth of rubber gasket can leak away all the energy saved with the expensive compressor. If designers and engineers kept the whole system in mind when designing products, this could be avoided.
What do we mean when we say “keep the whole system in mind” with respect to designing products? It means thinking about how the entire system of a product works as a whole, rather than focusing on its individual parts.
Now let’s take a closer look at what this means for a refrigerator. Keeping our food cool and well preserved is one of the great improvements of modern life. However, refrigeration is also the biggest energy user in most people’s homes, after heating and lighting. Refrigerators require 4-7% of US residential energy use, according to the Department of Energy; for a single appliance, that’s enormous. The cost of electricity over a refrigerator’s life often exceeds the purchase cost. Consequently, refrigerators demand more observation.
EnergyStar-rated fridges today use up to 75% less energy than an average fridge from the early 1980’s, but there’s still a long way to go. Fridges today are still nowhere near the theoretical minimum.
Improving the efficiency of components is an incremental process that works, but it often gets exponentially more expensive the better components you use – and you hit a point of diminishing returns.
For dramatic improvements, we need technology that’s both radically more efficient and cheaper to buy.
Amory Lovins from Rocky Mountain Institute uses the term “tunneling through the cost barrier” to refer to efficiency innovations that radically reduce all costs for manufacturers and users. For example, several years ago a high school physics teacher in Australia, Tom Chalko, made a refrigerator that used 93% less energy than a similar-sized unit, and only cost $400.
For comparison, the best-in-class commercially available unit of that size at the time only used 17% less energy than the reference model and cost $2,300. How did Chalko do this? His approach was simple: he bought a top-opening chest freezer and replaced the thermostat with one from a refrigerator.
Since it was designed to be a freezer, it was better insulated. It also opened upwards, so its cool air didn’t spill out into the room and force it to heat new warm air. Arguably, the biggest limitation of Chalko’s fridge was its form factor, and the awkwardness of the open top. There’s clearly a middle ground to be gained, using these efficiency measures and designing for better usability. Instead of just incrementally improving components, Chalko thought about the refrigerator as a whole system, and was able to go after the biggest gains for the least cost.
Thinking things through
Looking closer at the refrigerator as a whole, you begin to realize how effective this thinking is from a design point of view. There’s no point in building a super-insulated refrigerator if a single component, such as an icemaker, sticks a metal tube through that insulation to become a superhighway of heat leakage. Is energy use even the largest environmental impact of a refrigerator? What about the manufacturing of the steel and the insulation, and the disposal at the end of its life?
All of these are important impact areas; the life cycle assessment of an average fridge would show the environmental impacts from energy used during the fridge’s life vastly outweigh the impacts from manufacturing, distribution, or disposal. This helps us set design priorities: in this case, they might indicate adding more materials, such as insulation, to achieve higher performance.
An even more radical refrigerator design harks back to the era of the root cellar. Olin College of Engineering undergraduate students Ben Chapman and Cory Dolphin worked with a family in Vermont to capture and store the cold of winter weather. They built a large walk-in refrigerator-freezer with roof vents that open to the outside cold in winter.
Thick insulated walls are lined with thousands of re-used 2-litre bottles, each filled with saltwater to freeze and store the cold. The saltwater freezes at a lower temperature than plain water, thus keeping the unit cooler in the summer. For communities with northern climates, this kind of passive technology can cut the environmental impacts of refrigeration to nearly zero.
System mapping as a jumpstart
With such enormous performance gains possible, how can you do this, too? We propose combining whole-systems and lifecycle thinking into a unified process for sustainable design. The first step is to map the entire system of the product or service: What are its sub-systems, and how do they connect? Is it a sub-system of something larger?
When, why, and with what do your users use it? What is the entire lifetime of the product? Where do environmental and social impacts occur in the system? Building this map is a creative process similar to brainstorming.
In the Autodesk Sustainability Workshop, we apply this to two examples: a clothes dryer and a refrigerator.
In the case of a refrigerator, we include all the components of a fridge and how they fit together: the cool area, the insulation all around it, the heat exchange coils both inside and outside, the compressor sitting underneath the cool area, etc.
The system map also includes opening and closing the door, because much energy is lost. The map includes the mining of materials, manufacturing, transportation, use, and disposal, but it also includes where the food comes from and where it goes after it leaves the fridge, because the food is the whole reason the fridge exists.
Once the whole system is mapped, life-cycle assessment or other quantitative tools can be used to find the causes of the biggest environmental impacts: is it toxins in manufacturing? Energy use during the product’s life? Packaging waste? For the fridge, energy use completely dominates. Once you know this, you can set your goals for improvement, in quantitative terms that sit alongside standard project goals like 50% less energy use, at 10% lower purchase cost.
Brainstorm
Next, you brainstorm on your goal, again venturing out past individual silos to the product’s whole system: what are alternatives to every component or step in the system? How can you skip steps entirely? You can be more thorough and creative when you use the whole-system map you made earlier as a jumping-off point.
For instance, you could have several ideas for rearranging the fridge’s components so they work more efficiently, like having the compressor on top so its waste heat doesn’t rise into the refrigerated area, or replacing the one main door with a slew of insulated drawers to avoid spilling cold air out.
Finally, once you have a bevy of brainstorm ideas, you need some way to judge them. Some of the crazier ideas you can throw out right away, like only growing food inside the fridge to avoid opening the door. But to judge the plausible ones, you once again use your quantitative analysis tools like life cycle assessment, with some astute guesswork to estimate the performance and impacts of your ideas.
For instance, having the heat sink outside in a cold climate will probably perform better than simply moving the compressor to the top of the fridge. This will help you narrow the field to just the few best options that are worth prototyping or the one single idea worth pursuing.
Using whole-systems thinking, especially when combined with life-cycle thinking, turns sustainability into an innovation tool rather than a burden.
Whole systems and life cycle thinking have already begun to help designers and engineers open doors to radically higher-performance products and services in many industries, from refrigerators to buildings. There will be more opportunities in engineering to adopt this approach going forward, as we continue to try and decrease the demand for energy worldwide.
www.autodesk.com/sustainabilityworkshop
Interdisciplinary designer Dawn Danby, recognized by Fast Company in 2009 as one of the 100 Most Creative People in Business, has spent 12 years working in sustainable design.
Her projects have ranged from a tree-covered pedestrian bridge on the US-Canada border to closed-loop manufacturing strategies, furniture design and food distribution projects. She currently works with Autodesk’s Sustainability team, which seeks to integrate ecological and human impact considerations into the practice of 10 million designers, architects, and engineers worldwide who use their tools. Her latest initiative, the Autodesk Sustainability Workshop, provides free, lightweight videos and resources online to teach young engineers and designers the principles and practice of sustainable design.
Dawn co-authored Worldchanging: A User’s Guide to the 21st Century. She speaks regularly at events such as TEDGlobal and IDSA, was recognized as a Rising Talent by the Women’s Global Forum of Economy and Society, and won a fellowship with the Royal Society of Arts (UK). She has an industrial design degree from the Rhode Island School of Design and an MBA in Sustainable Business from the Bainbridge Graduate Institute.
Follow Dawn on Twitter @ecoworkshop (Autodesk) and @altissima (non-Autodesk)
Jeremy Faludi (LEED AP) is a sustainable design strategist and researcher. He has taught green design at Stanford University as well as teaching at California College of the Arts and Minneapolis College of Art and Design. He was sustainability research manager for Project FROG, the leader in modular commercial green buildings; he has also worked for Rocky Mountain Institute and Lawrence Berkeley National Labs, among others: www.faludidesign.com
Sustainability can be an innovation tool, say Dawn Danby and Jeremy Faludi
No
Default